Lupine Publishers- Latest Trends in Textile and Fashion Designing
Abstract
Textile and fashion industry is the thirstiest for water as it is one
of the largest water consumers from fibre production to finished
product. Unfortunately, all the scientific reports and opinions of
renowned water experts are predicting the severe water scarcity in
the near future in many parts of world particularly the developing
countries where textile and fashion industry production activities
are located because of cheap labour and less stringent environmental
laws. The gloomy picture of water scarcity is good enough to
caution the textile and fashion industry to take appropriate measures to
use the water resources sustainably and make all efforts to
save water at every stage of supply chain.
In the first part of this series attempt has been made to provide a
critical review of water distribution on earth, inequitable water
distribution in different regions of world and predictions on the
dangers of water scarcity which the humanity is going to face in
the near future. In order to face the situation of water scarcity
scientific community is working on developing concepts and tools
for sustainable water management. In this first part of the series, the
development of virtual water and water footprint concepts
have been highlighted. Water footprint concept is further elaborated
with water footprint analysis, pointing out difference between
virtual water and water footprint concept and how these two concepts are
used synonymously, comparison of water footprint
concept with carbon footprint, ecological footprint and life cycle
analysis concepts, environment impact and strategies to reduce
environment impact, criticism on water footprint concept and the various
other tools developed for sustainable water management
and certification and labelling. The subsequent parts of the series will
highlight the water footprint of textile and fashion industry
from fibre production to finished product stages.
Keywords: Water distribution on earth; Climate change; Water scarcity; Virtual water; Water footprint; Direct and indirect water
footprints; Water footprint analysis
Introduction
Many organizations worldwide are recognizing that water is
an essential ingredient in their business operations and the lack of
access to sufficient water quantities and water pollution are posing
a material risk to a growing number of companies [1]. Concern
about water is highlighted by a survey of the companies conducted
by the World Economic Forum in 2015, which indicated that there
will be serious water crises risk over the next 10 years [2].
According to World Business Council for Sustainable
Development, water is not only key to the world’s stability,
prosperity and peace but it is also essential for business to operate.
In the next three decades the demand for water will increase by 40-
50% for global food sector, by 50- 70% for municipal and industrial
sector and by 85% for the energy sector. Increasing competition for
water demands need immediate action, and radical change in the
way that companies manage water [3]. The textile industry touches
everyone’s lives through clothing, fabrics and other products;
however, it has a significant impact on the world’s resources.
Its production depends heavily on water, from raw materials
production to the industrial processing stages. Rising competition
for water is already impacting the textile and Equally significant is
the situation of workers in the supply chain, where in developing
countries working conditions often do not meet minimum human
rights and fair labor standards [4].
Cotton is the most liked fiber in textile industry. However, it
is the biggest culprit of water consumption and water pollution.
Despite only occupying 2.4% of World’s cropland, cotton accounts
for 24% of the world’s insecticide use and 11% of pesticides. Toxic
chemicals washing into waterways and entering the ecosystems,
is becoming a major source of pollution, especially in developing
countries [1]. Unsustainable cotton farming has resulted in the loss
of Aral Sea in central Asia. In the 1960s, the Aral Sea was the fourthlargest
lake in the world. It was an important source of life for the
surrounding communities and home to millions of fish. It now
covers a mere 10% of its original area. The local Uzbek communities
have suffered the loss of livelihoods and food sources while gaining
new health impacts. The dust from the lake is carcinogenic covering
most of the villages [5].
It’s estimated that around 20% of industrial water pollution
in the world comes from the treatment and dyeing of textiles, and
about 8,000 synthetic chemicals are used to turn raw materials into
textiles. Each year, textile companies discharge millions of litres
of chemically infected water into waterways. It is estimated that
a single mill can use 200 litres of fresh water per kilogram fabric
during chemical processing operations. So, there is not only highwater
consumption, but the chemicals pollute the water causing
both environmental damage and spreading diseases throughout
developing communities
In the developing world, where the majority of textile
manufacturing takes place, factories and textile mills are located
directly along or close by waterways such as rivers and canals.
These factories on an average use 1.5 billion cubic meters of fresh
water each year. In places like Dhaka in Bangladesh and Tirupur
in India the water is so polluted at times, it is not even safe for
livestock. Many industries and households that rely on fishing and
farming to make a living are now suffering as a result of the lack of
freshwater [6]. Another source of water pollution which has been
recently identified is the domestic washing of clothes. The wash
water is found to contain large number of micro fibers of synthetic
garments particularly polyester. These micro fibers from wash
water are eventually found to end up in sea endangering aquatic life
and eventually entering food system consumed by human being [7].
The present series of articles will highlight how textile and
fashion industry along its supply chain is thirsty of water which
is posing threat to the depleting sources of water worldwide
particularly in developing countries where most of the textile
and fashion production activities are concentrated because of
cheap labour and less stringent environmental laws. This first
introductory part of series highlights the water distribution on
earth indicating how very small quantity of fresh water is available
for human consumption though almost 71% area of earth is covered
with water. There is also non-equitable distribution of water in
different regions and water pollution is posing a serious threat to
already depleting water sources. This part of series also highlights
the development of water footprint concept, its importance, and
assessment of water footprints, strategies for reduction of water
footprints and certification and labelling. Subsequent parts of the
series will highlight water footprints from fibre to finished product
stages.
Water Distributions on Earth
The surface area of the Earth is 510 million square kilometers.
The Earth is a water heavy planet, so, if one breaks its surface area
into water and land segments, it would be:
a) Land 149 million km2
b) water 361 million km2 [8]
Following pictorial view depicts how earth is surrounded by
oceans (Figure 1).
About 71% area of earth is covered by water and 29% is the
land area. For this reason, earth is also known as blue planet. Water
distribution on earth is shown in the form of pie chart in Figure 2
[10]. It can be seen that about 97% of all water is in the oceans and
3% exists as fresh water. The middle pie shows the distribution of
that three percent of all Earth’s fresh water. The majority of fresh
water (about 70 percent), is locked up in glaciers and icecaps,
mainly in Greenland and Antarctica. This is equivalent to 2% of
total fresh water. This means that 99% of water available on earth
(ocean + ice caps, glaciers) is not usable [10]. The remaining 1% of
fresh water is available in two forms viz: surface water in the form
of rivers and lakes and ground water. The distribution of this 1% of
useable water is shown in Figure 3.
It can roughly be estimated that about 99% of all water (oceans,
ice caps, glaciers, saline water other than ocean, and atmospheric
vapors) is not available for our uses. Even of the remaining fraction
of 1% which is available for use is present in the form of surface
water (rivers and lake 1% and ground water 99%) [11].
Estimate of Global Water Distribution
Table 1 summarizes in terms of percent the distribution of all
the water available on earth. It can be seen that the world’s total
water supply of about 332.5 million cubic miles (1,386 million
cubic kilometers) of water, over 96 percent is saline, and of the total
freshwater, over 68 percent is locked up in ice and glaciers. Another
30 percent of freshwater is in the ground [12]. Thus, surface- water
sources (such as rivers and lakes) only constitute about 22,300
cubic miles (93,100 cubic kilometers), which is about 1/700th of
one percent of total water, yet rivers are the source of most of the
water people use.
Regional Inequity in Water Distribution
There are considerable variations in water availability in
different parts of world. While some countries are water rich, others
are at risk of becoming arid and may in the future be incapable of
sustaining their populations. Early in the new millennium, the top
five water rich countries were French Guiana, Iceland, Guyana,
Suriname, and Congo. At the bottom of the scale were Kuwait, the
Gaza Strip, the United Arab Emirates, the Bahamas, and Qatar [13].
Between those extremes a growing number of countries suffer
from various forms of water stress, either caused by the drying up
of resources, the depletion of the water table, the pollution of rivers
and lakes, or, as in Africa, years of drought. Over half the countries
of the world have been deemed either “dangerously dry” or “under
the poverty line of water availability” [14]. North America is
particularly fortunate to be home to the largest source of surface
freshwater on Earth, the Great Lakes Basin, containing about 21%
of the world’s total supply of fresh water [15]. By contrast, there
is a significant part of the world is arid, making up large parts of
Australia, Africa, and Asia. The so-called “hot spot” areas that are
running short of potable water include Northern China, large areas
of Asia and Africa, the Middle East, Australia, the Midwestern United
States and sections of South America and Mexico” [16].
India is depleting its groundwater so rapidly that its future
food crops and drinking water supplies are threatened [17]. With
the world’s second largest population, water shortages in India will
continue to have a massive impact. Ironically, there is considerable
rainfall in many regions of India, but it can be irregular or
unpredictable and Indian farmers have been at the mercy of rain
[17]. Unusual weather patterns in recent years, have afflicted India
with the competing “curses of drought and floods.” The escalating
problems are blamed on climate change [18].
Climate Change
In simple term “Climate change is characterized by erratic
weather patterns leading to droughts, floods, more frequent and
more intense natural disasters and other phenomena” [19]. A
volume of information is available on the effects of climate change
on environment including water scarcity. The purpose of this paper
is not to review the literature but only to mention the opinions
of some of the experts on the global effects of climate change on
water availability. Some of these opinions are quoted below:
Climate change “is going to affect the amount of water that comes
in as precipitation [20]. By one estimate, just two degrees rise in
temperature can adversely impact water accessibility for 3 billion
people [21].
José Graziano da Silva, Director-General of the FAO, commented
that “more extreme and frequent droughts resulting from climate
change are having devastating food security impacts, especially in
the most vulnerable regions of the world” [22]. While rainfall has
for centuries replenished the freshwater supply, given the current
levels of usage, precipitation will not suffice to meet the need and
demand. “Precipitation is a key source of water supply . . . but this
will only be useful if it can be caught and utilised effectively” [23].
Peter Gleick explained the connection between climate change and
the hydrological cycle: “Global climate change is going to have very
dramatic impacts on water resources because the hydrologic cycle
is such a fundamental part of the climate cycle” [24].
It has correctly been said that “water will be the vehicle through
which climate change is felt” [25]. Jouni Paavola of the Centre for
Social and Economic Research on the Global Environment at the
University of East Anglia commented that “climate change, water
and poverty are intimately intertwined in the developing world
because climate change will manifest itself primarily through
variation in the availability of water” [26]. According to UNO, the
effects of water scarcity on humanity are quite frightening It is
estimated that:
a) Presently around 700 million people in 43 countries
suffer from water scarcity.
b) By 2025, 1.8 billion people living in countries or regions
with absolute water scarcity and two- thirds of world’s
population could be living under water stressed conditions.
c) With the existing climate change scenario, almost half the
world’s population will be living in areas of high stress by 2030.
d) Sub-Saharan Africa has the largest number of waterstressed
countries of any region [27].
In spite of all the scientific controversies surrounding the issue
of climate change and despite the years-long political wrangling
that has delayed implementation of the Convention on Climate
Change, Nature seems to be taking matters into its own hands to
show humanity that it can no longer tolerate so much abuse. The
result is that natural disasters are becoming commonplace. Speak
to any senior citizen and one hears about the fact that in the past
such disasters were less frequent and not as terrifying as they
appear to be now. Such evidence may be anecdotal but there do
seem to be great extremes of climate causing havoc in many parts of
the world. Textile and fashion industry are the thirstiest for water
as it is one of the largest water consumers from fibre production to
finished product.
The above gloomy picture of water scarcity is good enough
to caution the textile and fashion industry to take appropriate
measures to use the water resources sustainably and make all
efforts to save water at every stage of supply chain. The present
series of articles will highlight the water footprints of textile and
fashion industry from fibre to finished products.
Virtual Water and Water Footprint Concepts
Virtual Water
Professor John Allen from King’s College London was the first
to introduce the concept of virtual water in 1994 [28]. It was based
upon analyses by Israeli water experts who found that it seemed to
make more sense for their arid country to import water-intensive
goods than to cultivate them themselves. Allen introduced the
virtual water concept, to throw light on how Middle Eastern
countries’ water requirements have exceeded available resources
since 1970. Trying to explain why there no war over water has been
when many economies in the Middle East region have only half the
water they need, Allan argued that economic systems solved the
water supply problem for the region, supplying water via trade. In
other words, water scarcity in these countries was being managed
by importing virtual water in the form of agricultural products from
the international market [29].
Allan stated: “The water is said to be virtual” because; the final
product contains only a small fraction of water compared to the total
volume of water actually used for its production. This means that
once the agriculture product is grown, the real water used to grow
it is no longer actually present in the product [30]. Virtual water
can be defined as the water that is required for manufacturing a
product or for rendering a service. Virtual water also contains the
actual amount of water that exists in certain product as moisture.
In everyday language, ‘virtual’ is often misinterpreted as
‘fictitious’ or as the opposite to ‘real’. As used here, however, ‘virtual’
is a notional quality that exists in its effects rather than in a physical
sense.
The concept of ‘embedded water’ (which was coined before
1994) has not managed to gain acceptance [31].
It is a revolutionary concept because it describes something
never conceptualized before. Thus, virtual water helps to
understand how virtual water flows between countries or regions
in the form of international trade [29]. The global trade in goods has
allowed countries with limited water resources to rely on the water
resources in other countries to meet the needs of their inhabitants.
As food and other products are traded internationally, their water
footprint follows them in the form of virtual water [32].
Two different definitions have been suggested in the attempt to
provide a more detailed description of virtual water [33]: According
to the first definition, virtual water denotes the water that is actually
required for manufacturing a certain quantity of a product at the
respective site of production (production-site definition) According
to the second definition, it is defined as the water that would be
necessary for manufacturing the same amount of the same product
at the place where the product is eventually needed (consumptionsite
definition)[34]. The First definition refers to the producer’s
perspective, while the second refers to the consumer perspective.
The amount of water needed for the production of agricultural
goods as well as industrial goods may considerably depend on the
production site. For example, let us assume that the amount of water required for manufacturing the same amount of the same
product is three times higher in country A than in country B. In
this case, the export of the same amount of this product from A to
B would involve a threefold transfer of virtual water compared to
an export from B to A. Consequently, product transfer from A to B
implies that A loses three times the amount of virtual water that B
would save if it produced the same amount of the same product in
its own country [31].
Importance of Virtual Water Concept
As nations work toward securing food, water, energy and
other essential inputs for people’s wellbeing, livelihoods and the
country’s economic development, most countries rely on imports
as well as exports of goods and services. A country may aim to be
self-sufficient by relying primarily on goods that can be produced
within its borders. Or a country may choose to reduce the burden
on the natural resources within its borders by importing water
intensive products from other countries.
For water-scarce countries it can sometimes be attractive to
import virtual water (through import of water-intensive products),
thus relieving the pressure on the domestic water resources. This
happens, for example, in Mediterranean countries, the Middle East
and Mexico. It results from protection of their domestic water
resources, land availability and land uses. Countries can both
import and export virtual water through their international trade
relations. Globally, the major gross virtual water exporters are USA,
China, India, Brazil, Argentina, Canada, Australia, Indonesia, France
and Germany. The biggest net virtual water importers are North
Africa and the Middle East, Mexico, Europe, Japan and South Korea.
There are many examples of water-poor countries that save
their domestic water resources by importing water-intensive
goods. Mexico, for example, imports maize and, in doing so, it saves
12 billion cubic meters per year of its national water resources.
This is the volume of water that it would need domestically if it had
to produce the imported maize within the country [32].
Merits of Virtual Water
Virtual water has major impacts on global trade policy and
research, especially in water-scarce regions, and has redefined
discourse in water policy and management. By explaining how and
why nations such as the US, Argentina and Brazil ‘export’ billions
of litres of water each year, while others like Japan, Egypt and Italy
‘import’ billions of litres of water each year
The virtual water concept has opened the door to more
productive water use. National, regional and global water and food
security can be enhanced when water intensive commodities are
traded from places where they are economically viable to produce
to places where they are not. While studying water scarcity in the
Middle East, Professor Allan developed the theory of using virtual
water import, via food, as an alternative water “source” to reduce
pressure on the scarcely available domestic water resources there
and in other water-short regions [35].
Limitations of Virtual Water [36]
Key shortcomings of virtual water concept are:
a) It relies on an assumption that all sources of water,
whether in the form of rainfall or provided through an irrigation
system, are of equal value.
b) It implicitly assumes that water that would be released
by reducing a high-water use activity would necessarily be
available for use in a less water-intensive activity.
c) It fails as an indicator of environmental harm and does not
provide any indication of whether water resources are being
used within sustainable extraction limits. The use of virtual
water estimates therefore offer no guidance for policy makers
seeking to ensure that environmental objectives are being met.
Water Footprint Concept
Building upon the concept of virtual water, the concept of
water footprint was coined in 2002, by Arjen Hoekstra, Professor in
water management at the University of Twente, Netherlands, and
co-founder and scientific director of the Water Footprint Network,
whilst working at the UNESCO- IHE Institute for Water Education,
to measure the amount of water consumed and polluted to produce
goods and services along their full supply chain [37].The water
footprint along the supply chain can be illustrated by taking an
example of the supply chain of product produced from cotton as
shown in Figure 4.
Supply Chain of Cotton Product
As shown in Figure 4 the water footprint is an indicator of
freshwater use that looks not only at direct water use of a consumer
or producer, but also at the indirect water use during the supply
chain. The water footprint of a product is the volume of direct and
indirect freshwater used to produce the product, measured over
the full supply chain [39].
It should be understood that the term ‘water consumption’
used in the concept of water footprint means the evaporation of
water from the surface or evapotranspiration of water from plants
and the water present in the product itself. This is based on the fact
that the agricultural or industrial products do not consume water
during their production except that present in the product itself
as natural moisture content. Percolation/run-off of water during
production to ground which results into ground water recharging
is excluded from the water consumption [40]. The two components
of water footprint i.e direct water and indirect water use is further
illustrated in Figure 5.
Figure 5: Schematic representations of the direct and indirect components of a water footprint [38].
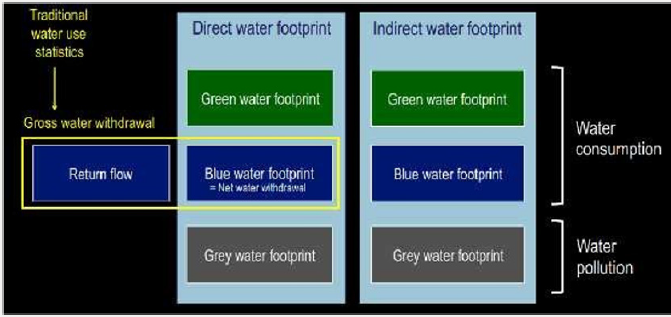
It shows that the non-consumptive part of water withdrawals
(the return flow) is not part of the water footprint. It also shows
that, contrary to the measure of ‘water withdrawal’, the ‘water
footprint’ includes green and grey water and the indirect water-use
components.
Hoekstra [41] differentiates between the footprint of a
consumer and a business as follows:
a) The water footprint of a consumer = Σ direct water use
(e.g. drinking) + indirect water use (production and services)
b) The water footprint of a business = Σ direct water use (e.g.
production) + indirect water use (supply chain).
The water footprint thus offers a better and wider perspective
on how a consumer or producer relates to the use of freshwater
systems. It is a volumetric measure of water consumption
and pollution. It is not a measure of the severity of the local
environmental impact of water consumption and pollution [42].
Earlier water-footprint studies were limited to the quantification
of resource use, i.e. the use of groundwater, surface water and soil
water [33,37,43]. Subsequently the water footprint concept was
further extended through quantifying the impacts of pollution as
well. As shown in Figure 6.
Water quality has been decided by quantifying the dilution
water volumes [44] required to dilute waste flows to such an
extent that the quantity of pollutants remain below agreed water
quality standards [45]. The most elaborate publications on how to
estimate water footprints are 2004 report on the Water footprint
of nations from UNESCO-IHE [46], the 2008 book Globalization of
Water [47], and the 2011 manual the water footprint assessment
manual: Setting the global standard [42]. Cooperation between
global leading institutions in the field has led to the establishment
of the Water Footprint Network in 2008.
Water Footprint of a Nation
The water footprint of a nation is defined as the total amount
of water that is used to produce the goods and services consumed
by the inhabitants of the nation. The water footprint of a nation has
two components. The internal water footprint defined as the water
use within the country in so far it is used to produce goods and
services consumed by the national population. The external
water footprint of a country is defined as the annual volume of
water resources used in other countries to produce goods and
services imported into and consumed in the country considered
[48,49]. The water footprint of a nation is illustrated by following
two Figures 7 & 8.
The national water footprint is composed of domestic goods and
services plus imports (incoming water) minus exports (outgoing
water). Each of these has green/blue/grey components. Data are
from Hoekstra & Mekonnen (and show global averages to illustrate
differences in water composition [50]. It will also be interesting to
know the Global average numbers and composition of all national
water footprints, internal and external as shown in Figure 9.
Figure 9: Global average numbers and composition of all national water footprints, internal and external [51].
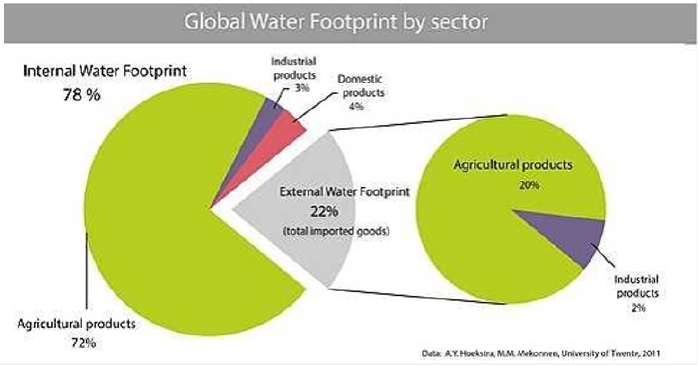
The external part of a nation’s water footprint varies strongly
from country to country. Some African nations, such as Sudan, Mali,
Nigeria, Ethiopia, Malawi and Chad have hardly any external water
footprint, simply because they have little import. Some European
countries on the other hand, e.g. Italy, Germany, the UK and the
Netherlands have external water footprints that constitute 50-80%
of their total water footprint. The agricultural products that on
average contribute most to the external water footprints of nations
are: bovine meat, soybean, wheat, cocoa, rice, cotton and maize
[51].
Components or Colors of WF
While determining the total water footprint, the consumption
of rain water, ground water, surface water along with extent of
water pollution is taken into account. These are considered as
components of water footprint and are represented by Green water
(Rain water or precipitation), Blue water (ground and surface
water) and Grey water (volume of fresh water required to dilute
the pollutants). The total water footprint is the summation of these
three colors of water. In case of national water footprint, the import
and export of virtual water is also taken into account [52].
Green Water Footprint
Green water resources have been defined as the infiltrated
rainfall in the unsaturated soil layer [53]. The green water
footprint therefore refers to the precipitation consumed by a
crop through evaporation from soil (surface evaporation) and
evapotranspiration from plants and moisture stored in the soil
(also known as effective or productive precipitation) [54]. Green
water is particularly relevant for agricultural, horticultural and
forestry products. In general, the utilization of “green” water does
not apply to industrially or commercially manufactured products
and services [47].
Blue water footprint
Is water that has been sourced from surface or groundwater
sources. Irrigated agriculture, industry and domestic water use
can each have a blue water footprint. The share of “blue” water
contained in these products is defined as the share of the utilised
water that is released into the atmosphere as a consequence of
evaporation or sublimation and evapotranspiration (in case of
agriculture products) and since it cannot be expected to return to
the region considered, it may be regarded as consumed. The same
applies to service provisions and the water used for domestic
purposes. The term blue water consumption is different than blue
water withdrawal. A comprehensive definition of the blue water
footprint is that it includes all irrigation water and any direct water
use in industry or in households, minus return of water in the
ground [55]. The green and blue water footprint in relation to water
balance of catchment area is shown in Figure 10.
For understanding freshwater appropriation by humans in
relation to the hydrological cycle, one can consider a river basin.
Other terms for ‘river basin’ often used are ‘catchment area’,
‘drainage basin’, ‘drainage area’ and ‘watershed’. The total annual
water availability in a catchment area is given by the annual volume
of precipitation. The total annual precipitation flow will leave the
basin again, partly through evapotranspiration and partly through
run-off from the catchment. Both the evaporative flow and the
run-off can be appropriated by humans. The green water footprint
refers to the human use of the evaporative flow from the land
surface, mostly for growing crops or production forest. The blue
water footprint refers to the consumptive use of the run-off flow,
in other words, the abstraction of run-off from the catchment in so
far as it does not return to the catchment in the form of return flow
[42].
Grey Water Footprint
The grey component of a water footprint is defined as the volume
of freshwater required in receiving water bodies for the assimilation
of any pollutant resulting from production so that acceptable water
quality standards are met [54]. Thus, the grey water footprint is an
estimated measure of the potential water pollution caused by the
production of agriculture and non- agriculture products and supply
chain. The grey water footprint considers point- source pollution
discharged to a freshwater resource directly through a pipe or
indirectly through runoff or leaching from the soil, impervious
surfaces, or other diffuse sources [52].
In case of products other than agriculture, the dilution volume
is determined in relation to the concentration of contaminant
which needs highest volume of water to bring the contaminant
concentration to the acceptable level [31]. Whereas in case of
agriculture runoff, the contaminant concentration is generally
calculated by assuming that 10% of all nitrogen fertilizer applied
to a crop is lost via leaching and taking the average nitrogen
application rate by crop in the country being assessed; the assumed
fraction of nitrogen leachate is then divided by the maximum
acceptable concentration of nitrogen in the receiving water body.
The consideration of grey water is relatively new in water
footprint and was not included in earlier water footprint studies,
such as Chapagain and Hoekstra [43] it was introduced by
Chapagain et al. [56] using the term “dilution water” which later
evolved into the concept of grey water [56]. By including all three
components, the water footprint aspires to encompass all kinds of
direct and indirect consumptive (blue and green) water use and
pollution assimilation (through grey water estimates).
In case of agriculture products, Blue and green water footprints
are calculated by multiplying the modelled volume of blue and green
water use (m3/output unit e.g. m3/ton). In this way, any rainfall
used in situ by the crop (green water) is distinguished within the
water footprint of a product, as well as the volume of irrigation
(blue) water assumed to be applied to each crop. The grey water
footprint is an estimate of the amount of water needed to assimilate
nutrients in agriculture water runoff to ground and surface water.
Difference between Virtual Water and Water Footprint
The water footprint refers to the water used to make a product.
In this context there is no difference between virtual water and
water footprint. However, the water footprint is a multidimensional
indicator, not only referring to a water volume used, but also making
explicit where the water footprint is located, what source of water is
used, and when the water is used. Thus, the most typical feature of
water footprint is the importance of specifying space and time. This
is necessary because the availability of water highly varies in space
(regions) and time, so that water appropriation should always be
considered in its local context.
Other applications of the water footprint are for example
identifying hotspot areas of the water footprints of certain products,
consumer groups or businesses, and formulating response
strategies to mitigate water footprint impacts. This additional
information is crucial in order to assess the impacts of the water
footprint of a product. The term virtual water is used mainly in case
of international trade for importing or exporting the product. e.g.
when an agriculture or commodity product is imported this means
that along with the imported product one is importing virtual water
which was required to produce the product in the country of origin
and vice- versa for the export of the product [57].
Velázquez et al. [58] expressed that virtual water is defined
as a physical indicator of the amount of water needed to produce
goods and services, and water footprint as the amount of water
needed to produce the goods and services that will be consumed by
a country or an individual. Therefore, virtual water is consolidated
as a production perspective indicator while water footprint is
a consumption perspective indicator. This difference between
virtual water and water footprint is interesting since it allows us
to profit from two indicators defined from different perspectives
and capable of identifying the subjects who are responsible for
water consumption, whether producers or consumers. Despite
the evident difference between the two concepts there could be
a growing confusion surrounding both concepts. They have often
been used as synonyms and, thus, have partly lost the potential that
is associated to the difference between them. But the relevance of
these two concepts is so significant that both public administrations
and big corporations (Danone, Nike, Levi-Strauss, Coca-Cola, etc.)
have decided to explore the possibilities of including them to
support various alternatives in their decision- making processes
[59].
Criticism of the Water Footprint Concept
The most important criticism of water footprint is its weak
relationship between the footprint’s indicator and the footprint’s
environmental impact. The blue and green components in particular
measure resource use and not environmental impact. A crop may
have a large water footprint but little environmental impact, as it is
growing in a water-abundant environment, whereas a crop with a
lower water footprint may have a significant environmental impact
by using scarce blue water supplies. To compare the environmental
friendliness of products, it is of little value to compare their water
footprint indicators. This especially applies to the green water
component, usually the major component of a water footprint
indicator.
Evaporation of rainwater and evapotranspiration from plants are
natural processes of the hydrological cycle and evapotranspiration
from natural vegetation may even exceed evapotranspiration from
crops. Therefore, correcting for the evapotranspiration from natural
vegetation, seems to be more appropriate if environmental impacts
are considered [60]. By taking green water into account in a water
footprint assessment to detect hot spots is a questionable method.
In this respect, the Water Footprint Network’s Assessment manual
states that the green water footprint in relation to sustainability
needs further research and it recommends excluding a quantitative
assessment of green water scarcity from practical policy- making
for the time being [42]. Nevertheless, considering the green water
content of water-intensive commodities can be useful in the
context of resource use and international trade. In such a case,
green water is looked at as a scarce resource and not as a source of
environmental damage [50].
Grey Water
There is no clarity about grey water measurements. Green
water in case of agriculture and blue water can be quantified but
it is problematic to add the share of grey water while determining
the total water footprint indicator because part of grey water can
be brought into system by recycling after effluent treatment. In fact,
in the zero-discharge principle almost 80-90% of water is recycled
except which is lost in the form of sludge and evaporation. Also, in
many countries grey water is used in agriculture [39].
Berger and Finkbeiner [61] criticized the attempt to combine
water quantity and quality aspects in one single indicator and
the way water pollution is converted into volumes of grey water.
Also, the lack of reliable data to actually calculate water footprint
indicators is a source of criticism. Water quality is less elaborated
than water quantity in the water footprint concept and databases.
The immense variety of pollutants, their use and behaviour make it
a laborious task to include water quality aspects in water footprint
assessments [62]
Finally, it is argued that the full water footprint assessment
provides nothing that is not already provided by existing water
resource management and supply chain assessment tools [63,64].
The water footprint concept would be one of sticking new labels on
old concepts. However, one may also argue that there is added value
in bringing together existing tools for water resource management
and supply chain management to form one complete approach,
because it connects two different worlds of researchers and users,
which may be fruitful for new insights.
Development in Water Footprint Methodology
According to the Water Footprint Assessment Manual of the
Water Footprint Network [42] the goal of assessing water footprints
is to analyze how human consumption relates to the issues of water
scarcity and pollution, and to see how consumption can become
more sustainable from a water perspective. Human consumption
is linked to freshwater use and the emission of pollutants to water
along production and supply chains of consumer products and their use. The tool to analyze water footprints is what is called a
‘water footprint assessment’.
During the first few years of the development of the water
footprint concept (2002–2008), the focus was on calculating the
footprint’s total size. This resulted, for instance, in the notion that
one apple costs 125 litres of water and one cotton shirt about 2500
litres [40]. These large volumes of water connected to products
captured the imagination of many, and NGOs have used them to raise
awareness among consumers, producers and governments about
the size and global dimensions of water use related to consumer
products. The location of the footprint components and their
geographic context received little attention. From 2008 onwards,
more attention has been given to the location of footprints and their
geographic context. The water footprint theory has evolved from an
accounting method for calculating an indicator to a methodology to
perform water footprint assessments in which the accounting phase
is followed by an impact or sustainability assessment. An early
example of such an assessment is the study by Van Oel et al. [65]
that relates the water footprint indicators for Dutch consumption
to locations with water scarcity. This development has also directed
the research by the Water footprint network towards the mapping
of global water scarcity and pollution [66, 67].
It should be noted that the developments around the water
footprint concept have resulted in some confusion about the
footprint’s exact meaning. Originally, the water footprint was
synonymous with its total volume, resulting in the well-known
water volumes assigned to products. However, currently, and in
the context of a water footprint sustainability assessment, it is
considered a geographically explicit indicator, representing not only
water-use volumes and pollution, but also showing the locations
of the footprint. This is logical, as a water footprint sustainability
assessment focuses on the water footprint components in different
catchments and sub-catchments, and not on the total size of
the water footprint. Confusion about the definition of the water
footprint also has been created by various Water footprint network
publications and in particular by the assessment manual, which
uses both definitions side by side.
Water Footprint Assessment
A full water footprint assessment as described in the Water
Footprint Network manual consists of four phases as shown in
Figure 11 Studies on virtual water trade and studies carried out to
estimate the water footprint indicator for products only; typically
consist of the first two phases. When the focus is on the impacts
related to the water footprint, the assessment will comprise the
first three or all four phases [40].
Setting Goals and Scope
A water footprint study can be undertaken by nation, river basin
authority and business organization for many different reasons.
For example, a national government may be interested in knowing
its dependency on foreign water resources or it may be interested
to know the sustainability of water use in the areas where waterintensive
import products originate. A river basin authority may
be interested to know whether the aggregated water footprint of
human activities within the basin violates environmental flow
requirements or water quality standards at any time. The river
basin authority may also want to know to what extent scarce water
resources in the basin are allocated to low-value export crops. A
company may be interested to know its dependence on scarce water
resources in its supply chain or how it can contribute to lower the
impacts on water systems throughout its supply chain and within
its own operations [40].
Water Footprint Accounting
The main goal of the accounting phase is to keep record of
green, blue and grey water volumes associated with the production
processes and the supply chain till the product reaches to the
consumers. These data are used to calculate the aggregated water
footprint indicator by adding up all recorded water volumes. They
can also be used to calculate water footprint components such
as the green, blue and grey water footprint components, or the
imported ‘virtual’ water from various countries as components
of the water footprint indicator for national consumption. When
calculated for a particular product while ignoring product use,
the water footprint indicator is also referred to as the ‘virtual’
water content of the product. Depending on the type of study,
the water footprint accounting phase could also be the basis for a
sustainability assessment [42].
Water Footprint Sustainability Assessment
It may be carried out from the point of view of
a) Water sustainability
b) Social or human sustainability
c) Economic sustainability
Water Sustainability
The main goal of a water footprint sustainability assessment is
to trace processes along the production and supply chains or those
associated with product use, which are located in unsustainable
‘hot spot’ areas. A hot spot is a location where production process
uses water from overexploited water resources, pollutes water
above water quality standards or where water allocation and use
are considered unfair or economically inefficient. To trace hot
spots, the major water footprint components are viewed in their
geographic context, preferably at catchment or sub- catchment
level.
The catchment areas concerned are assessed on sustainable
water use, taking into account all major claims on water resources
and emissions to water within the catchment or sub- catchment
areas. If the required data are not available, separate catchment
studies should be done. A catchment’s possible environmental
unsustainability is determined by the degree of blue and green
water scarcity and the water pollution level, taking into account
temporal (time related) aspects, as problems of water scarcity and
pollution may be concentrated around certain periods of the year.
Social Unsustainability
Social unsustainability is considered to the case when basic
human needs are not being met for all people in the catchment or
sub- catchment area or when basic rules of fairness are not being
applied for water- related issues.
Economic Unsustainability
Economic unsustainability would refer to a situation where
water is neither allocated nor used in an economically efficient way.
The temporal (time related) aspect of water scarcity is becoming
more important as climate change may influence precipitation
patterns as well as total amounts.
Theory, methods and tools of a full water footprint assessment
as well as its practical application are still developing and in an
experimental phase [42].
Response Formulation
To support response formulation with respect to the detected
hot spots and water-inefficient processes, the Water footprint
network manual gives an overview of response options for various
actors, including consumers, companies, farmers, investors and
governments. On a global scale, for instance, water-intensive
production processes would best be located in water-abundant
areas, and optimal use could be made of rain-fed agriculture to
release the pressure on water-scarce areas. On a local scale, one
could think of technical measures to reduce water use and pollution,
and optimization of water allocation according to fairness or
economic criteria.
Assessment Tools
To reduce the impacts of water footprints through sustainable
water management, the first step would be to assess current water
management practices, associated impacts and risks, and response
options. To that end, a number of tools have been developed
from different perspectives such as sustainable corporate water
management, sustainable water management in production and
supply chains, and sustainable management of drainage basins.
Below, the most relevant tools are identified:
Water Footprint Network
The website of the Water Footprint Network presents several
tools and many publications; for instance, The Water Footprint
Assessment Manual, calculators for individual water footprints,
national water footprint case studies and a database. The
methodology described in the manual can be applied to various
types of water footprint assessments, including those on product
and company level. The Water Stat database contains data from
various studies on water footprints, virtual water, water scarcity
and pollution. The Water Footprint Assessment Tool is free online
web application assisting users in water footprint quantification,
sustainability assessment and response formulation.
Eureaka
Under its 7th Framework Programme for Research and
Technological Development, the EU has funded the One Planet
Economy Network project (OPEN:EU). This work resulted in the
free to use Internet tool EUREAKA, providing integrated ecological,
carbon and water footprint data [69,70]. The tool models the flow
of goods and services between 45 countries and regions, covering
the global economy for 57 individual sectors. The sectors cover a
range from agricultural and manufacturing industries to transport,
recreational, health and financial services.
Global Water Tool
The Global Water Tool (GWT), launched in 2007, is a free to use
tool from the World Business Council for Sustainable Development
(WBCSD) for companies operating in multiple countries to map
their water use and assess risks for their global operations and
production and supply chains [71]. It does not provide specific
guidance on local situations that require more in-depth systematic
analysis. The tool was developed under the leadership of WBCSD
member and global engineering company CH2M HILL. An advisory
board of 21 other global companies provided guidance in pilot
testing. Expertise was provided by The Nature Conservancy and
the Global Reporting Initiative. More than 300 corporations have
used the tool which is regularly updated with improved data sets
and functionalities.
Local Water Tool
The Local Water Tool (LWT), launched in March 2012, is
a free to use tool from the Global Environmental Management
Initiative (GEMI) that will help companies conduct systematic
assessments of their relationship to water in order to create sitespecific
sustainable water management strategies [72,73]. GEMI is
a non-profit organization of 26 companies dedicated to fostering
environmental, health and safety and environmental sustainability.
The development of the tool was carried out in cooperation with the
WBCSD to link the tool to the Global Water Tool. It is also supported
by an additional number of participants like AkzoNobel and Shell.
Aqua Gauge
The Ceres Aqua Gauge is a free to use tool that allows companies
in the management of water risks [74]. Production, supply chains
as well as the impacts of product use on water are considered.
Ceres is a US-based coalition of investors, environmental groups,
and other public organisations working with companies to address
sustainability challenges, such as climate change and water scarcity.
The tool was developed by Ceres in collaboration with the World
Business Council for Sustainable Development (WBCSD), Irbaris,
and the IRRC Institute.Input was provided by representatives from
over 50 financial institutions, companies and NGOs, among others
are Robeco Asset Management, WWF and The Nature Conservancy
Water Risk Filter
The Water Risk Filter is an online application hosted by WWF
which is designed to help companies and investors to assess water
risks and give guidance on how to respond to such risks [75].
The application aims to cover all relevant aspects of water risks:
physical risks (water scarcity, water pollution, ecosystem threats,
dependency on hydropower) including those in the production and
supply chain, regulatory risks and reputational risks. These risks
comprise those that are basin related and company- related
Risk levels are determined according the scores on a set of
indicators multiplied by a corresponding weighting. The scores
come from a facility-specific questionnaire or are derived from a
number of global data sources, including blue water scarcity and
water footprint data from the Water Footprint Network.
The application has been developed for WWF in collaboration
with DEG KWF Bankengruppe.
Corporate Water Gauge
The Corporate Water Gauge is a tool of the Centre for Sustainable
Organizations (CSO) providing an indicator for measuring the
ecological sustainability of an organization’s water use, using a
watershed-centric approach [76]. Supply chain aspects are not
considered. The tool is not available for free. Licences are granted to
clients who engage CSO to either provide related training or assist
with at least one application at a site of their choosing.
Aqueduct
The World Resources Institute (WRI) is developing the Aqueduct
Water Risk Atlas from which a first version is now available
for free [77,78]. At the heart of Aqueduct is a global database of
water risk information that will enable companies, investors,
governments and others to create detailed water risk maps. An
Aqueduct Alliance, including the Netherlands Ministry of Foreign
Affairs, supports the project financially and expert advisors from
companies, government agencies, NGOs and academia participate
in Aqueduct Alliance Working Groups.
GWP Toolbox
The toolbox developed by the Global Water Partnership is
a free and open database to support integrated water resource
management with a library of background papers, policy briefs,
technical briefs and perspective papers as well as huge sections
of case studies and references [79]. The toolbox pays only limited
attention to supply chain aspects and the water footprint concept.
Charting Our Water Future
Charting Our Water Future is a report of the Water Resources
Group on the possibilities to close the growing gap between (blue)
water supply and demand by the 2030 [80]. The report provides an
analytical framework to facilitate decision-making and investment
in measures increasing supply and improving water productivity.
As a key tool, the ‘water-marginal cost curve’ is presented, which
provides a microeconomic analysis of the cost and potential of a
range of existing technical measures to close the projected gap
between demand and supply within a particular basin area. The
report offers case studies from four countries with drastically
different water issues together with an extensive list of assessed
measures.
Relationship Between Water, Carbon and Ecological Footprints
A “footprint” in general has become known as a quantitative
measure showing the appropriation of natural resources or pressure
on the environment by human beings. The water-footprint concept
is part of a larger family of concepts that have been developed in
the environmental sciences over the past decade. From this point
of view water footprint is analogous to carbon and ecological footprints. Methodologically there are many similarities between
the different footprints, but each has its own peculiarities related
to the uniqueness of the substance considered [81]. The features
of water, carbon ecological and nitrogen footprints are shown in
Figure 12. Recently, the nitrogen footprint was introduced as a tool
to measure the amount of nitrogen released into the environment
in relation to consumption [82].
The carbon footprint measures the amount of greenhouse
gases produced, measured as carbon dioxide equivalents (in
tons). The water footprint measures water use (in cubic meters
per year). Carbon footprint addresses the issue of climate change,
the water footprint relates to the issue of freshwater scarcity. In
both cases, a supply-chain perspective is promoted. However, for
a carbon emission it doesn’t matter where it happens, but for a
water footprint it does matter. A carbon emission at one place can
be offset by carbon emission reduction or sequestration in another
place, which is not true for water footprint. One cannot reduce the
local impact of water use in one place by saving water in another
place.
Ecological Footprint
Ecological footprint was introduced in the 1990s which
denotes the area (hectares) needed to sustain a population, the
water footprint represents the water volume (cubic meters per
year) required [83-85]
About ten years later a water footprint concept was launched
to measure the human appropriation of the globe’s freshwater
resources. Although both concepts have different roots and
measuring methods differ in some respects, the two concepts have
in common that they translate human consumption into natural
resource use. The ecological footprint measures everything in use
of space (in hectares), whereas the WF measures the total use of
freshwater resources (in cubic meters per year) [86]. The three
indicators are complementary, since they measure completely
different things. Most typical for the water footprint is the
importance of specifying space (region) and time. This is necessary
because the availability of water highly varies in space and time, so
that water appropriation should always be considered in its local
context [57].
Water Footprints in Life Cycle Assessments
Life Cycle Assessment (LCA) is a methodology for assessing
the environmental performance of a product or service over its life
cycle from cradle to grave and addresses basically all environmental
impacts caused by the use of fossil fuels and other natural
resources and of the emission of harmful substances to air, water
and soil. Elementary materials and energy flows are quantified in
a Life Cycle Inventory (LCI), while environmental impacts from
resource use and emissions are quantified in a Life Cycle Impact
Assessments (LCIA) for ‘impact categories’ such as ozone depletion,
climate change, acidification and eutrophication, and higher-level
impact categories such as human health, ecosystem quality and
resource depletion.
In addition to these quantifications of overall environmental
impact, LCIA also allows for the detection of environmental hot
spots in production and supply chains, as a basis for optimising
the environmental performance of a particular product or service.
This all makes LCA a potential tool for assessing the environmental
sustainability of products regarding water also and thus it is
a potential alternative to the Water footprint network’s water
footprint approach. However, traditionally only the grey water
footprint is covered by LCA, through the assessment of the emission
of harmful substances to water according to impact categories such
as eutrophication and aquatic toxicity. Until recently, water use was
largely neglected in LCAs. Methods for taking the impacts of blue
water use into account are now being developed, while green water
is hardly considered at all [61,87-89].
Many types of damages, such as acidification or toxicological
or eco- toxicological impacts on humans and ecosystems, often
are regional or local impacts, making it important to evaluate
them in their geographic context. In current LCA approaches, such
non-global impacts are often calculated on a continent or country
basis. Regionalization is therefore recognised as an important step
towards improving the accuracy and precision of LCA results. As
also the assessment of water use requires regional distinction, the
current research on the integration of blue water use in LCAs takes
regionalization into account. The impact of blue water use is often
represented by a water withdrawal or consumption to availability
ratio in a catchment area, so water from a water body that is
overexploited
would have a higher weighting than water from one that
is under-utilized.
In May 2012, a new operational LCA method was launched
called the ‘IMPACT World+’. This method addresses both water
use and regionalization [90-92]. The method assesses worldwide
impacts of blue water consumption on a watershed level on aspects
such as human health and aquatic and terrestrial ecosystem quality.
Together with the calculation of regionalized impacts of emissions
to water, using traditional LCA impact categories, the new method
addresses blue water scarcity as well as water pollution in a
spatially differentiated way.
The LCA approach to water footprints is also adopted in the
development of the ISO standard 14046, which is to provide a
specification of requirements and guidelines to assess and report
on the water footprints of products, processes and organisations,
based on LCAs [93-95]. However, it is difficult to predict the extent
to which recent developments of the integration of water footprint
indicators in LCAs may lead to practicable tools that could, for
instance, be used by companies to assess their water footprints and
to detect the unsustainable hot spots along their production and
supply chains.
Groundwater Footprint
Gleeson et al. [96] have launched the ‘groundwater footprint’
concept, which reveals the water balance of global aquifers.
According to this concept, the water balance between groundwater
aquifer inflows and outflows is converted to a surface area size
which is called the ‘groundwater footprint’ and can be compared to
the surface area of the groundwater aquifer.
Groundwater stress occurs if the footprint area is larger than
the area of the aquifer itself and varies in severity depending on the
ratio between the area of the footprint and the area of the aquifer.
The footprint only addresses ‘hydrologically active’ aquifers that are
replenished, and therefore does not include fossil groundwater. The
groundwater footprint concept does not relate groundwater use to
consumption of products via production and supply chains. Gleeson
et al. conclude that at least 20% of the world’s ‘active’ aquifers are
overexploited – some of them heavily and that currently 1.7 billion
people live in areas with groundwater stress.
This new concept can complement the water footprint
sustainability assessment that focuses on water balances of
catchment areas. Groundwater aquifers have different geographical
boundaries from those of catchment areas, although they are
hydrologically related to these areas. Water stress in catchment
areas may cause groundwater depletion. Therefore, there is a large
geographical overlap between catchment areas with water stress
and groundwater aquifers with water stress. The groundwater
footprint approach is useful for water footprint assessments as it
maps areas with groundwater scarcity in addition to catchment
areas with water scarcity. This also implies that the source of
the water used (surface water, shallow groundwater or deep
groundwater) is relevant information to determine the scope of the
environmental impact.
Environmental Impacts of a Water Footprint
The main environmental impacts related to a water footprint
are listed below:
a) For blue water, the environmental impacts will be in the
form of polluted water discharges to river, reduced water levels
in rivers and lakes, reduced groundwater levels, and a reduced
capacity to assimilate pollutants emitted to water. This, in turn,
would lead to a reduced availability of good quality blue water to
other users and the ecosystem.
b) For green water, when it is used by water-intensive crops
evaporating more than the natural vegetation, the environmental
impacts may be a reduced run-off and infiltration, leading to a
reduced availability of blue water with effects as described above.
c) For the emission of pollutants to water, the environmental
impacts may consist of the pollution of surface water and
groundwater, leading to human health risks and possible
degradation of ecosystem.
All these impacts, together, lead to problems of water quantity
and quality, and impact on humans and ecosystem worldwide.
According to the OECD Environmental Outlook to 2050 [97]
increasing water demand will exacerbate water stress in many
river basins, particularly in densely populated areas in rapidly
developing economies. More river basins are projected to come
under severe water stress by 2050. The number of people living in
stressed river basins is expected to increase from 1.6 billion in 2000
to 3.9 billion by 2050, equalling over 40% of the world population
by then, almost the entire population of South Asia and the Middle
East and large shares of the population of North China.
In many regions of the world, groundwater is being exploited
faster than it can be replenished, leading to increasing groundwater
depletion. Gleeson et al. [96] estimated that the size of the global
groundwater footprint is currently about 3.5 times the actual area
of aquifers, and that about 1.7 billion people live in areas where
groundwater resources are under threat. It is found that over 20%
of groundwater aquifers are overexploited. The quality of surface
water is expected to deteriorate in the coming decades, caused by
different groups of pollutants, such as micro-pollutants, pesticides
and nutrient overloads from agriculture and poor wastewater
treatment of industrial wastes.
The OECD Environmental Outlook to 2050 [97] assessed
the consequences of nutrient overloads, which were found to
be increased eutrophication, biodiversity loss and disease. For
example, the number of lakes at risk of harmful algal blooms is
expected to increase by 20% in the first half of this century. The
occurrence, frequency, duration and extent of oxygen depletion and
harmful algal blooms in coastal zones are projected to increase as
rivers discharge rapidly increasing amounts of nutrients into the
sea. Harmful algal blooms may damage ecosystems, fish stocks and
fishery opportunities, and pose a danger to human health.
A water footprint assessment does not cover all water-related
problems connected to production processes, as it only addresses
water use in volumes and water pollution. For instance, the impact
of production processes on river discharge patterns and flooding,
and the morphological changes, such as dams and canalisations, are
not assessed. Hoekstra et al. [66] developed a method to include
temporal aspects in their comparisons between blue water use and
water availability at a monthly basis for the world’s major river
basins. Effects of climate change on availability, in time and place,
of green and blue water could be included in a water footprint
sustainability assessment.
Furthermore, the issue of people lacking access to safe drinking
water and adequate sanitation, is not addressed in the water
footprint approach, since this often does not concern physical
(blue) water scarcity, but economic water scarcity [98,99].
Strategies to Reduce Environmental Impact
The severity of the environmental impact related to a water
footprint component depends on the size of the footprint and its
spatial and temporal context. It is determined by the contribution
of the footprint component to water stress or pollution in the
related catchment and sub-catchment areas compared to the
overall degree of water stress or pollution. Often, multiple users
are responsible for water stress and pollution in those areas; this
implies that reducing the size of those footprint components related
to water stressed or polluted areas often is not enough to solve the
existing water problems. To achieve a significant reduction in water
stress or pollution in catchment and sub-catchment areas, often a
more comprehensive approach is needed, involving all major water
users in those areas. The impacts related to a water footprint may
be reduced along the following complementing strategies as shown
in Figure 13.
Options to reduce the negative impacts related to a water
footprint can be found in all steps along the production and
supply chains and all the players have to play their roles. Some of
the measures which can be adopted by consumers/community,
business organizations and government agencies are outlined
below [57].
Consumers/Community
Application of WF concept is important for consumers to use
water economically in order to avoid the unnecessary wastage of
water. Some of the following measures for water reduction are worth
implementing. It is necessary to make the distinction between direct
and indirect water footprints. Direct water footprint is that where
the consumer is using water for daily needs like drinking, cooking
food, flushing toilets, bathing, laundry, etc.; whereas indirect use
encompasses many things. Just to give an example drinking coffee.
In this example direct water use will involve water used for making
a cup of coffee, whereas indirect water use means water used for
cultivation of coffee. Some of the measures that could be adopted
by consumer are:
Direct Water Footprint
Installation of water saving toilets, water-saving showerhead,
closing the tap during teeth brushing, using less water in the garden
and by not disposing medicines, paints or other pollutants through
the sink. These measures will fall in the category of reduction in
direct water footprint.
Indirect Water Footprint
The indirect water footprint of a consumer is generally much
larger than the direct one. A consumer has basically two options
to reduce indirect water footprint. One option is to substitute a
consumer product that has a large water footprint by a product
that has a smaller water footprint. Examples: eat less meat or
become vegetarian, drink tea instead of coffee, or even better drink
plain water. Not wearing cotton but artificial fibre clothes saves lot
of water. The second option is to stick to the same consumption
pattern but to select the cotton, beef or coffee that has a relatively
low water footprint or that has its footprint in an area that doesn’t
have high water scarcity. However, this is possible when consumers
have proper information to make that choice. Since this information
is generally not available in the world of today, an important
thing consumer can do now is to ask product transparency from
businesses and regulation from governments. When information is
available on the impacts of a certain article on the water system,
consumers can make conscious choices about what they buy [40].
Business
For business organizations the concept of water footprint is
important for the following reasons.
a) Environmental awareness and strategy to protect the
environment is often part of ‘corporate social responsibility’.
Reducing the water footprint can be part of the environmental
strategy of a business, just like reducing the carbon footprint.
b) Many businesses actually face serious risks related to
freshwater shortage in their operations or supply chain e.g. how
can a company in jean manufacturing survive without continued
supply of water to the cotton fields?
c) To meet government regulations on water usage and
water pollution standards.
d) Incorporation of water footprint strategy may be
considered as an opportunity to reinforce the corporate image or to
strengthen the brand name.
In case of business the direct water footprint will involve water
consumption during production whereas indirect WF will be water
consumption during supply chain. Businesses can reduce their direct
water footprint by saving water during production and bringing
water pollution to zero by using the strategy of reduce, recycle and
treat before disposal. WF analysis is therefore important to achieve
Zero discharge during production. Indirect WF during supply chain
is not under the control of business, therefore, may be difficult to
achieve. However, by making agreements with their suppliers with
certain standards can help to reduce supply chain water footprint
and making supply chain fully transparent to consumers. Among
the various alternative or supplementary tools that can help
improving transparency are: setting quantitative water-footprint
reduction targets, benchmarking, product labelling, certification
and water footprint reporting [40].
Government Agencies
Water scarcity is posing threats particularly in many developing
countries. This is mainly due to fact that governments are not aware
the principles of water management. It is time that Government in
each country frame rules and regulations and set policies for water
conservation at national level. WF concept is therefore important
for any government agencies. Governments should engage with
consumers and businesses to work towards sustainable consumer
products. National water footprint accounting should be a standard
component in national water statistics and provide a basis to
formulate a national water plan and river basin plans that are
coherent with national trade policy and national environmental
policy.
Water Footprint Certification and Labelling
Certification and labelling are potential instruments to
promote sustainable water use and to reduce the impact related
to the water footprint of business organisations, products and
consumers. Certification and labelling schemes are aimed to provide assurance that certain criteria are being met, with respect
to production methods and product characteristics. This also
includes schemes that operate within a supply chain – business to
business–and those aimed at communicating product quality to
consumers. Certification and labelling are essential communicative
tools to create awareness along the supply chain, consumers and
government authorities.
Many existing certification and labelling schemes address
environmental issues, but only a few include water use, and none
cover the full life cycle of a product to reflect the total impact on
water footprint. There is currently no single scheme that addresses
all of the water issues that would need to be covered. According
to Richter [100] there are two main reasons for a company to be
involved in water certification:
a) To encourage water efficiency and good water
management;
b) To support the corporate reputation regarding social
responsibility and sustainability
Certifying Organization
Alliance for Water Stewardship the Alliance for Water
Stewardship is developing the International Water Stewardship
Standard, a performance standard that can be used globally to
certify certain water users who voluntarily practice sustainable
water management [101-103]. The standard is aimed at companies
that use significant amounts of water in their operations. To qualify
for certification, applicants will be required to measure their direct
and indirect water consumption and other physical and chemical
characteristics in the drainage basins along their production
and supply chains. The standard should not disadvantage small
and medium-sized enterprises or enterprises in least developed
countries.
This means that any application of the standard should be
both practical and feasible. The AWS is following the ISEAL Code of
Good Practices, which is aimed to ensure that voluntary standards
are effective and accessible and bring about positive social,
environmental and economic effects. AWS intends to align the
reporting component of its standard with indicators of the Global
Reporting Initiative to the greatest extent possible.
European Water Partenership
Similar to the development of the International Water
Stewardship Standard, the European Water Partnership has
developed a European Water Stewardship (EWS) standard for
businesses and agriculture to assess, verify and communicate
sustainable water management practices at operational and river
basin levels [104-106]. The standard is valid on a global scale but
based on local assessments with a focus on Europe. Supply-chain
and water footprint aspects are covered by criterion 4.2 of the
current version of the standard, stating that ‘Water management
in the supply chain shall be evaluated on long term.’ and that ‘The
purchase of products and material from water sustainable suppliers
shall be achieved’.
Conclusion
Many authoritative scientific reports and opinions of experts in
water management studies point out the grave situation of water
scarcity world is going to face in the near future. This will be further
exasperated by inequitable regional distribution of water in many
parts of world. The other problem associated with water scarcity is
the water pollution and for various reasons this has reached to an
alarming level in some parts of world. Since water is essential for
all human activities and to maintain the eco-system it is time that
everyone considers water as a precious commodity and manage its
use in a sustainable way.
Among the various tools developed for sustainable water
management, virtual water and water footprint concepts are
gaining popularity. The virtual water concept is more suited for
application in international trade where it is emphasized that one
of the ways to face the problem of water scarcity is to import the
water intensive products from water rich countries. The water
footprint concept is applied in local context for sustainable water
management.
There is a correlation between water footprint, carbon
footprint and ecological footprint except the parameters considered
are different in each concept. Water footprint emphasizes on
quantitative water use in terms of different evaporative processes.
The environmental impact in water footprint concept is correlated
with the grey water expressed in terms of dilution water required
to assimilate the polluting impurities to the acceptable standard.
However, this has been criticized based on two facts firstly, in many
countries grey water is used for agriculture and secondly many
industries particularly the textile and fashion industry are following
the concept of zero water discharge for recycling of polluted water.
It is expected that as the water footprint concept further develops,
the criticism related to the accounting of environmental impact
would become clearer.
For more Open Access Latest Trends in Textile and Fashion Designing Please Click Here:
Follow on Linkedin : https://www.linkedin.com/company/lupinepublishers
Follow on Twitter : https://twitter.com/lupine_online
No comments:
Post a Comment